It is a basic and unavoidable fact in the antimatter business that in order to produce antihydrogen, antiprotons and positrons must be mixed. So, ALPHA must have the ability to confine and manipulate charged plasmas with reasonable efficiency and at cryogenic temperatures to boot!
This is accomplished in ALPHA through the use of Penning traps, a type of trap commonly used in plasma physics experiments to confine charged plasmas. Charge is in fact the difference, and indeed the dilemma faced when attempting to trap antihydrogen. Because antihydrogen is neutral, it cannot be held in a traditional Penning trap. This is where ALPHA’s unique magnetic trap comes in.
As for positron, antiproton and electron plasmas: a Penning trap will certainly do the trick. In a Penning trap, charged plasmas are confined in a combination of magnetic and electric fields.
Plasmas in a Penning trap are axially confined by quadratic electric potentials. In a perfect Penning trap, the potentials are produced by the application of voltages to equipotential surfaces shaped like hyperboloids of rotation. Radial, or transverse, confinement of particles in the trap is provided by a solenoidal magnetic field applied along the trap axis. Charged particles in the trap travel in the direction of the magnetic field lines and hence cannot escape outwards, or perpendicular to the field.
ALPHA uses a Penning trap variation called a Penning-Malmberg trap. The difference is that the electric potentials in a Penning-Malmberg trap are not perfectly quadratic. The potentials in ALPHA are produced by the application of DC voltages to a stack of hollow cylindrical electrodes, as opposed to hyperboloidal. It’s true that quadratic potentials would allow for a more straightforward analytical interpretation of particle motion in the trap. However, the Penning-Malmberg scheme in ALPHA is actually extremely effective in confining particles in sufficiently well shaped (if not perfectly quadratic) and is much more technically feasible.
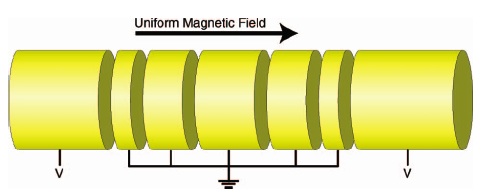
(Figure from Charlton et. al, "Antihydrogen for precision test in Physics" 2008.)
Figure 1. An illustration of a typical Penning Malmberg trap. voltages are applied to the two outer electrodes, forming a potential well. (Figure from Charlton et. al, "Antihydrogen for precision test in Physics" 2008.)
In the trap, particles are subjected to the Lorentz force. The Lorentz force deflects particles perpendicular to the magnetic field, causing them to spiral around magnetic field lines. The spiraling particles are also accelerating, and hence emitting radiation. This effect is called cyclotron radiation. Cyclotron radiation is important in ALPHA because the emission of radiation corresponds to the cooling of the particles themselves.
For positrons and electrons, this effect works fantastically: cyclotron radiation effectively cools the particles in a matter of seconds. Antiprotons, however, are far more massive. It would take antiprotons over 300 years of sitting in a 1 Tesla magnetic field to cool through cyclotron radiation alone! Needless to say, waiting so long is out of the question. Instead, ALPHA uses several additional tricks to bring antiprotons down to temperatures suitable for antihydrogen formation.